Canada has urgent and challenging energy choices to make. We will need to rapidly scale power generation to service the needs of a growing economy, while simultaneously reducing net-carbon emissions to zero by 2050 to meet our climate targets. Given the current technological outlook, there is no single energy source that can meet those competing demands. But one thing is clear: nuclear power can be a key part of that lower-emissions future — and an increasingly promising option is to commercialize small modular reactors (SMRs).
SMRs are more adaptable versions of today’s large-scale reactors and could solve many of the issues facing the nuclear industry. While most SMRs are still on the drawing board, they promise to reduce the costs of construction and operations, expand the range of applications across the economy, and potentially improve safety factors. If commercialized successfully, SMRs could bring new, non-emitting sources of electricity to big cities and remote communities, while providing flexibility to key Canadian industries that now power production processes with fossil fuels.
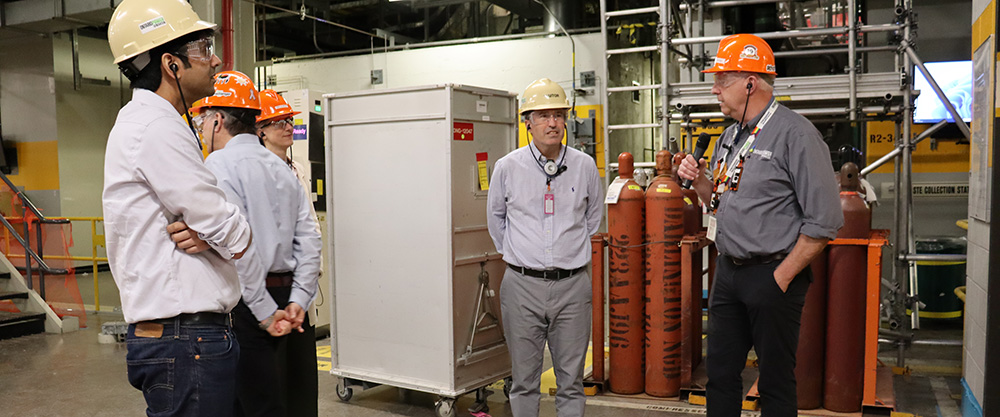
Vivan Sorab, Senior Manager, Clean Technology RBC (Left), John Stackhouse, SVP, Office of the CEO RBC (Middle), Chuck Lamers, Senior Communications Advisor (Right)
The repercussions of SMRs could be far-reaching, with the global SMR market projected to reach $150 billion to $300 billion annually by 20401. Given the country’s seven decades of success in nuclear energy, Canada starts from a position of strength. SMRs could revitalize Canada’s nuclear industry, allowing us to export our talent and proven expertise to a world that is committed to triple nuclear power by 20502. Several countries including the U.S. and Britain have announced major public-private partnerships to capture those opportunities.
Canada has already taken an early lead in deploying a new generation of SMRs. One such reactor—GE-Hitachi BWRX-300—is close to the start of construction at the Darlington Nuclear Generating Station east of Toronto. That single SMR, the first of four that will be built at Darlington, could eventually provide electricity for 300,000 homes3. Other SMRs in various stages of licensing across the country could eventually power industrial facilities and remote mines and replace diesel in isolated communities.
- Types of SMRs
SMRs vary in size, design and components, electrical and thermal outputs, and intended applications. Early SMRs will be used to generate electricity for cities, but the technology’s versatility could mean that scaled-down, or micro-SMRs, could eventually be used by industries, small, off-grid communities, and mines. Like large nuclear reactors, SMRs are broadly classified based on how they are cooled and the way their fission reactions are controlled.Light and heavy water reactors
The most established technology, use water to cool the reactor core and slow down neutrons – subatomic particles that help sustain nuclear fission reactions.High-temperature gas-cooled reactors
Uses gases like helium to cool the reactor core and have faster neutron speeds, allowing higher temperatures to be produced.Molten salt reactors
Use salts to cool the reactor core. They can operate at high temperatures and can use a range of fuel types.Fast neutron reactors
As their name suggests, fast neutron reactors use fast neutrons to trigger and sustain fission reactions, making reactors more fuel-efficient and increasing power production.
Source: US Department of Energy
To optimize the drive to net zero, Canada has formulated a national plan to develop and commercialize SMRs. In 2018, Ottawa created a coalition drawn from various levels of government, Indigenous communities, academia, power utilities and other industries to draw up a coordinated Small Modular Reactor Roadmap4. This was followed by an SMR Action Plan in 20205. To remain at the forefront of a potential SMR revolution, Canada must seek further ways to finance and regulate the development and commercialization of reactors. No one expects that will be straightforward. But an effective rollout of a nationwide plan to deploy SMRs promises an adaptable new energy source for the country, and a powerful catalyst for Canada’s transition to a greener economy.
Key findings
- Canada will need to build a projected 85 SMRs at a cost of $102 billion to $226 billion to reach our net-zero emissions target by 2050.
- SMRs could help power electrical grids, while their size and flexibility would allow them to replace fossil fuels in specific industrial processes and other off-grid settings.
- To ensure the country has the expertise to support the growth of an SMR industry, Canada will need more than 5000 full-time, skilled workers on average between 2025 and 2040.
- Indigenous partnerships and expertise will be a critical for the development of Canada’s SMR industry and its supply chains, from uranium mining to component manufacturing and eventually to new projects in areas on or near traditional Indigenous lands.
- With no uranium-enrichment facilities of its own, Canada will need to work with allied nations such as the U.S. and France to secure stable supplies of enriched nuclear fuel to deploy its SMR fleet.
What is an SMR?
Nuclear energy has been used to produce carbon-free electricity since the 1950s, providing stability and diversity to national power grids. In addition to large-scale plants, small, bespoke nuclear reactors have been used to power submarines, aircraft carriers and planetary spacecraft. Some small reactors have been installed inconspicuously for research in national laboratories or university campuses, like McMaster University in Hamilton6 and the Royal Military College of Canada in Kingston7.
Not all SMRs meet the 300MW criterion — and not all reactors smaller than 300MW are SMRs. Companies in Britain, for instance, are developing SMRs rated at 470MW capacity9, and India’s nuclear reactor fleet includes several reactors rated at 220MW that are not considered SMRs10.
Climate change has renewed interest in the nuclear industry, particularly SMRs. While there are an estimated 98 SMR designs around the world in various stages of development, only Russia and China are currently operating commercial SMRs. The majority of SMRs remain in the design phase. The U.S. has the most SMR designs under development, followed by Russia, China, Japan and Canada. Denmark, with no native nuclear power, is also pursuing the technology with a floating SMR design11.
SMR designs are being advanced by companies worldwide
Count of SMR designs in development
Source: World Nuclear Association, RBC Climate Action Institute
Although a handful of private players are working to commercialize SMRs, government support is key for the technology to scale. In the U.S., the Department of Energy and private companies have jointly invested over $1 billion in SMR development12. The Tennessee Valley Authority (TVA), the largest U.S. public utility, has thrown its weight behind SMR technology. As early as 2019, it obtained federal approval for SMRs at its Clinch River Nuclear site13. In November 2020, Britain announced a £215 million spending package to be matched by private investment14.
In 2022, Canada announced $29.6 million for research and supply chain frameworks, $70 million for research on minimizing SMR waste, and $51 million for the Canadian Nuclear Safety Commission to build regulatory capacity for SMRs15,16. In the same year, the Canada Infrastructure Bank provided $970 million in funding for the Darlington SMR17. New Brunswick invested $10 million in 2018 to establish a research cluster for SMRs18, and another $80 million in total in two advanced SMR companies19. Saskatchewan invested $80 million in a micro-SMR project in 202320.
To remain in the forefront of the potential SMR revolution, Canada will need to continue to refine the ways it finances and regulates the development and commercialization of the reactors.
A strategic moment for Canada
SMRs could be a significant part of Canada’s future energy mix. How big a share depends in part on how quickly SMRs can be developed and deployed. Given the current technological outlook at least, SMRs have several advantages compared to the other main energy sources.
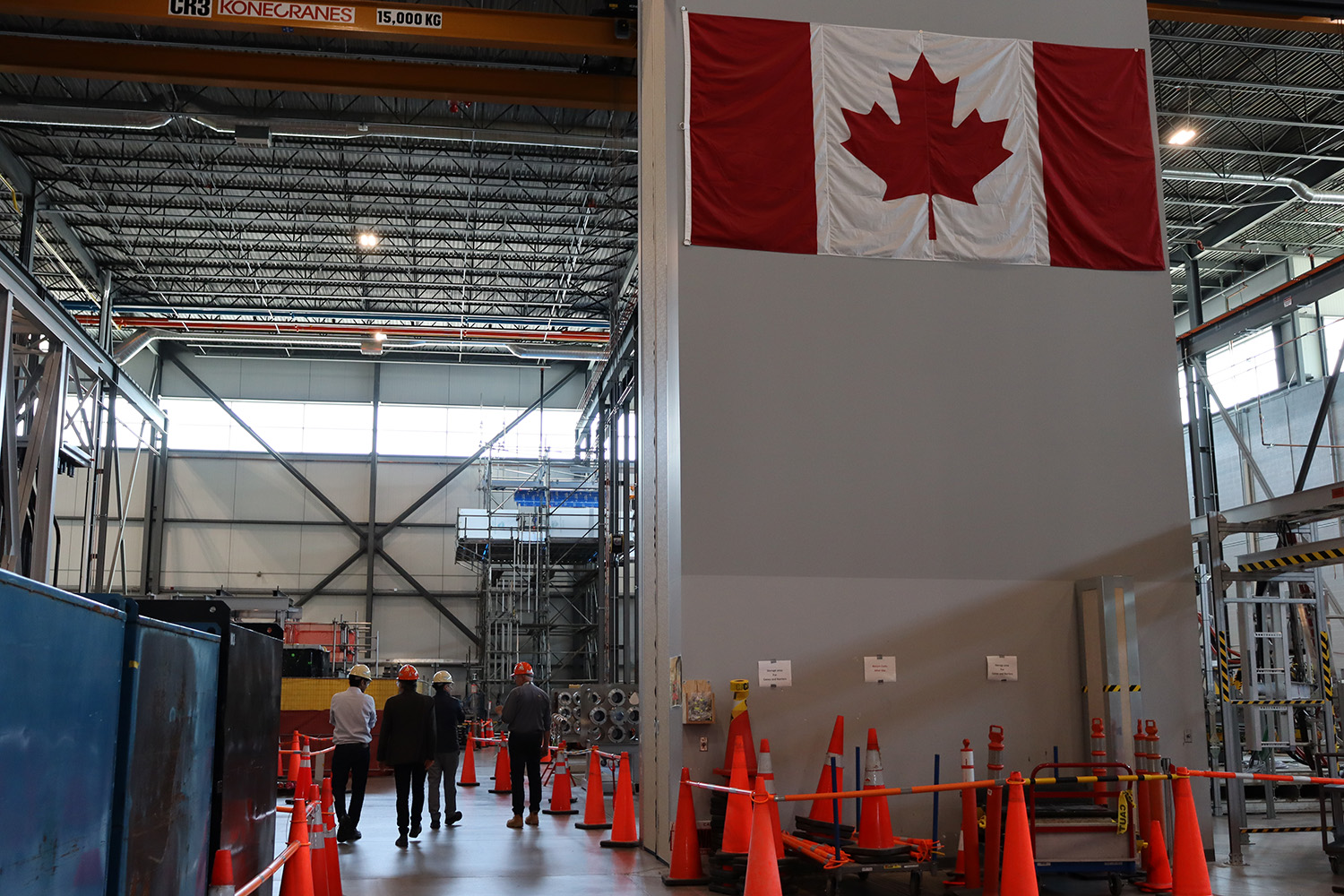
Hydroelectric projects have been a mainstay of the Canadian energy landscape, but big projects are not possible or viable in many parts of the country, their massive expense, land impact, and the lack of new, quality resources make the case for new dams challenging. Prolonged droughts may challenge their reliability.
Renewables such as wind and solar provide relatively inexpensive electricity compared to nuclear options. But that power is intermittent, dependent on when the sun shines and the wind blows, which means renewables generally need to be backstopped by expensive batteries or emissions-intensive natural gas.
Natural gas plants retrofitted or built with carbon capture and sequestration (CCS) could provide relatively clean and reliable power. But on the drive to net zero, they will largely be limited to geographies where emissions can be captured and stored underground (mostly Western Canada), in a process that often comes with a hefty price tag and uncertainties in economics and commercialization potential.
Nuclear energy, to reach its potential, must overcome a track record that has included cost overruns, long project timelines, and low social acceptance in parts of the country such as British Columbia and Nova Scotia. There also continue to be concerns around nuclear safety and waste management. Nuclear is the world’s second-highest source of zero-emissions power after hydroelectric dams but its share of global electricity production has dropped from 17% in the 1990s to 9% today (with natural gas, coal and renewables filling the gap21.)
SMRs will be the 5th largest source of power in a net-zero Canada
Installed capacity, MW
Source: Canada Energy Regulator, RBC Climate Action Institute
SMRs, if commercialized, could accelerate nuclear project timelines, lower costs, and bring nuclear to geographies with grids too small to accommodate large power plants. Under a net-zero scenario from the federal Canada Energy Regulator, the country will need 25 gigawatts of SMR capacity—equivalent to about 85 grid-scale SMRs—by 2050, which would provide 7% of Canada’s power capacity. Under that scenario, onshore wind would account for 30% of the total, hydro 26%, utility-scale solar 10%, abated natural gas 7% and large nuclear 3%22. By leveraging SMRs as a source of non-emitting power, Canada could save 41 megatons of emissions, on average, annually between 2030 and 2050 relative to unabated natural gas generation23.
SMR applications
Canada’s electricity supply is one of the world’s greenest, with 81% of its generation fed by hydro, nuclear, and wind and solar power24. But there is no easy solution that would decarbonize the 19% of Canada’s grid that still relies on fossil fuels.
Reducing the 75 megatons of CO2 equivalent emitted annually from Canada’s industrial sector26 is a net-zero imperative. SMRs can help Canadian industries decarbonize by providing uninterrupted, non-emitting electricity and heat to commodity producers. In the longer term, SMRs could be used to produce low-emissions hydrogen and synthetic fuels that may aid in the carbon-intensive steel, cement and petrochemical industries.
But deploying SMRs will be difficult in certain industrial processes given existing technologies. Steelmaking in blast furnaces and cement manufacturing require temperatures at or above 1,000 Celsius, which current SMR designs would not be able to produce27.
The mining sector produces 2% of national emissions28, but has made steady progress on decarbonization. For instance, nickel miners are converting their mine vehicle fleets to electric29 and pursuing projects that use tailings to capture CO2 30.
But complexity varies and some mines will be more challenging to decarbonize. Canada’s largest and heaviest carbon-emitting mines are the massive iron ore operations in Newfoundland and Labrador, Quebec and Nunavut. These operations will continue to rely on fossil fuels in the near-term because there are no alternatives at present that can produce the high temperatures (at least 1,300 C) they need to process ore31.
Decarbonizing this carbon-intensive sector is arguably Canada’s greatest climate challenge. Oil sands extraction is responsible for 12% of national emissions32, and consumes 30% of the Canada’s natural gas output33, which it burns in boilers to produce steam for in-situ production techniques.
The path ahead:
What Canada needs to go big on SMRs
Large nuclear power plants have a track record of going over budget during construction. Capital costs for large-scale nuclear plants in the U.S., France, Canada and Germany have escalated 60% to 200% since the 1970s35, and some recent projects exceeded their budgets by billions of dollars.36
SMRs could eventually reverse that trend—at least on paper. Lower design complexity, better safety features that may streamline regulation, and potential modular manufacturing and on-site assembly are all features that advocates say will help them overcome the industry’s cost problems. If SMRs can be built on time and on budget, they may be cost competitive with other sources of low- or non-emitting energy.
The SMR record is still nascent, and therefore difficult for capital markets to assess. There are only two SMRs operating commercially, one in Russia, the other in China; both saw cost escalations and project timeline delays37. An SMR in Argentina has been under construction since 2014. With only a handful of projects in advanced development around the world, it is unclear whether SMRs will achieve economies of scale and lower their costs in the near-term.
Building the 85 SMRs that Canada needs to reach our net-zero climate targets will cost a projected $102 billion to $226 billion38. Because of nuclear’s long lead times, that spending will have to begin soon. Under one net zero forward trajectory, Canada’s power sector will need 93% of Canada’s SMR capacity to be built before 204039. Capital spending to support that rapid growth in the 2030s will need to reach an average of $9 billion to $20 billion annually40.
Canada already has one advantage in place. The federal clean technology investment tax credit could offset 30% of the capital costs for SMRs. Based on current projections, the credits would lower the cost of electricity from SMRs by 24% while boosting their competitiveness41.
In building a new fleet of SMRs to generate clean power, Canada can plan the newest phase of its energy transition in concert with Indigenous communities at all stages of the SMR value chain from uranium mining through to project development and operation, and eventually spent fuel management.
Early signs have been encouraging. Indigenous groups are already seeking opportunities in Canada’s SMR buildout, and government funding has helped establish bodies such as the Indigenous Advisory Council to provide a unified national voice for Indigenous communities around SMRs42. In New Brunswick, the North Shore Mi’kmaq Tribal Council and its seven First Nation member communities signed equity agreements last year with Moltex Energy Canada and ARC Clean Technology Canada to develop and deploy advanced SMR technology. In Saskatchewan, three Indigenous-owned companies partnered in 2021 to jointly invest and build businesses to service SMR markets44.
Building out a successful national SMR industry could create a substantial export opportunity for a new generation of Canadian nuclear. Key areas of opportunity include licensing the GE-Hitachi BWRX-300 design, scheduled to be built at the Darlington nuclear plant. Provincial crown corporation Ontario Power Generation, the Tennessee Valley Authority electric utility and the Polish company Synthos Green Energy invested $400 million to bring the BWRX-300 design to completion45. Should this design scale, OPG would share technology licensing revenues from future BWRX-300 projects.
Canadian project management expertise will also be in demand. The emergence of a successful SMR industry could create a $3 billion to $10 billion annual opportunity for Canadian expertise by 2040 in pre-construction (e.g., land acquisition, environmental studies, permitting), and indirect services (e.g., engineering, project management, quality assurance, testing, and commissioning)46.
Export opportunities could extend to other parts of the SMR industry, such as uranium supply and conversion. Canada is the world’s second-largest uranium miner, after Kazakhstan—producing 15% of global uranium supply47. A tripling of global nuclear capacity by 2050, as projected at the COP28 UN Climate Change Conference in Dubai, would create significant opportunities for Canada’s uranium mining industry.
Uranium conversion—the processes that transform raw uranium ore to fuel- or enrichment-ready products—is another area of opportunity. Canada controls 28% of the world’s operating uranium conversion capacity, which is less than Russia (38%), but ahead of China (25%) and France (8%)48.
To streamline the introduction of SMRs, the private sector and the federal and provincial governments will have to overcome negative perceptions about nuclear energy. They will also face government restrictions in certain provinces: B.C. has a long-standing ban on nuclear power generation49, and Nova Scotia has only recently reopened the possibility of nuclear power generation in the province50.
Polls indicate Canadian societal attitudes to nuclear power are changing. Between 2012 and 2023, Canadian public support for nuclear power increased from 37% to 55%, with 62% now viewing it as essential to Canada’s net-zero strategy. A majority of the public in Ontario, Saskatchewan, and Alberta supports nuclear power, as do pluralities in Manitoba and the Atlantic provinces.
Public opinion polls indicate Canadians who may oppose a nuclear project deemed beneficial if it was built close to where they live are increasingly in the minority. Surveys that attempt to capture public concerns about the selection of new plants and nuclear waste management sites have indicated local opposition to new projects—including nuclear—peaked in 2011 and have been in decline ever since.
Other challenges persist. In 2022, 60% of Canadians said they had never heard of SMRs and another 25% said they were only vaguely aware of them. Skeptics were not convinced by the information about SMRs’ smaller footprint, affordability and enhanced safety, but were open to learning more51.
Canada will need a rapid revitalization of its nuclear workforce to support growth of the SMR industry in the coming decades. At least a third of the country’s nuclear professionals were approaching a retirement age in 2019. Ensuring that the country has the nuclear-critical skills it needs will be key, as 4,000 professionals across all trades are set to retire by 2025.
Canada needs a new workforce to build and operate its SMR fleet
Number of Workers
Source: Conference Board of Canada, NB Power, RBC Climate Action Institute
Building and operating the near-term SMR fleet in Ontario, Saskatchewan and New Brunswick, and Alberta will require an average of 5,300 workers between now and 2040, and about 2,400 to operate and maintain the fleet thereafter. To service domestic and international demand, associated sectors like uranium mining and processing will need to grow their workforces as well.
Because its CANDU reactor fleet runs on natural uranium, and an international treaty prevents the country from enriching uranium at home, Canada has not developed domestic uranium enrichment capacity53. Canada will need to look beyond its borders for enriched uranium to run a future nuclear fleet that will almost certainly need the fuel. The amount it requires will depend on what designs get developed, both in terms of SMRs and large nuclear power plants.
All but one of the SMR designs being commercialized in Canada today require uranium to be enriched to different degrees. Canada’s earliest SMRs will be fueled by Low Enriched Uranium (LEU), which will initially be sourced from France and the U.S54. More advanced designs will need High-Assay Low Enriched Uranium (HALEU).
Uranium enrichment is geographically concentrated. As of 2022, Russia controlled 40% of the world’s enrichment capacity55, and it is the only commercial producer of HALEU. Issues over accessing HALEU have already disrupted SMR projects in the U.S. As nuclear’s resurgence stagnant enrichment capacity, following years of oversupply and underinvestment, enriched uranium could become bottlenecked in the future. Addressing that bottleneck could become critical.
Spent nuclear fuel in Canada currently comes from the country’s CANDU reactor fleet and has been safely managed since the first commercial reactors began operating about five decades ago. As Canada commercializes SMR designs, new types of spent nuclear fuel will require long-term management. The physical properties, quantities and management protocols around spent fuel will vary significantly between different SMR designs. In some cases, spent fuel will be of a kind that is well understood and for which management protocols exist internationally. For others, new waste-management protocols will need to be developed.
SMRs could help decarbonize and expand electricity grids servicing large and small population centers and provide energy to beachhead industrial markets. But as electricity demand grows, traditional large nuclear power plants must continue to play a role. Proven conventional nuclear technologies, such as Canada’s home-grown CANDU reactor and potential alternative technologies from abroad, are well placed to provide additional non-emitting capacity.
With the exception of the Point Lepreau reactor in New Brunswick, Canada’s nuclear fleet is concentrated in Ontario. With the successful refurbishments of two units in Darlington almost half a year ahead of schedule, and commitments for additional refurbishments at the Pickering Nuclear Generating Station, Canada is set to keep the fleet running for at least another 30 years56. But translating this experience into new nuclear capacity will be essential if we are to reach its climate goals while maintaining secure energy supplies.
Preparing for the age of small
Canada has been a global leader in the peaceful use of nuclear energy for over 75 years. Early research at labs in Montreal and Chalk River helped lead to breakthroughs in the industry and development of the safe and versatile CANDU reactor technology, which has been used across eastern Canada and exported to six other countries. The Pickering, Bruce and Darlington nuclear generating stations have been strategic drivers through the 1990s, producing important supply chains in Ontario and employing tens of thousands of skilled workers. While fiscal tightening and global nuclear-safety fears arrested the industry’s growth in the 1980s and 1990s, decisions to reinvest in Bruce and Darlington have since brought the sector new life.
The promise of SMRs now presents Canada with new choices about our nuclear future. If SMRs can be developed and commercialized quickly and cost-effectively, they can help Canada meet growing demand for electricity and its commitment to reach Net Zero by 2050. But we will need to move faster. For Canada to achieve Net Zero emissions by 2050, 93% of SMR capacity must come online in the 2030s, more than twice as fast as Canada achieved its conventional nuclear capacity buildout between the 1970s and 1990s57.
The good news is that Canada is taking an early lead in deploying SMRs. The GE-Hitachi BWRX-300 prototype is nearing construction at Darlington, while other SMRs are in various stages of licensing. Success could unlock a new source of energy for non-emitting baseload power for Canada’s grids, and off-grid power for remote locations. Success will also position Canada to be an important exporter of SMR components and expertise.
Canada will need to be nimble. Nuclear power is by far our most complicated source of electricity. And the commercialization of advanced approaches to nuclear, through SMRs, will require a diverse mix of capital, skills, fuel supplies and public policy. That, in turn, will require a coordinated national approach to make this potentially transformative technology a key part of our energy future.
Related Reading
For more, go to rbc.com/climate.
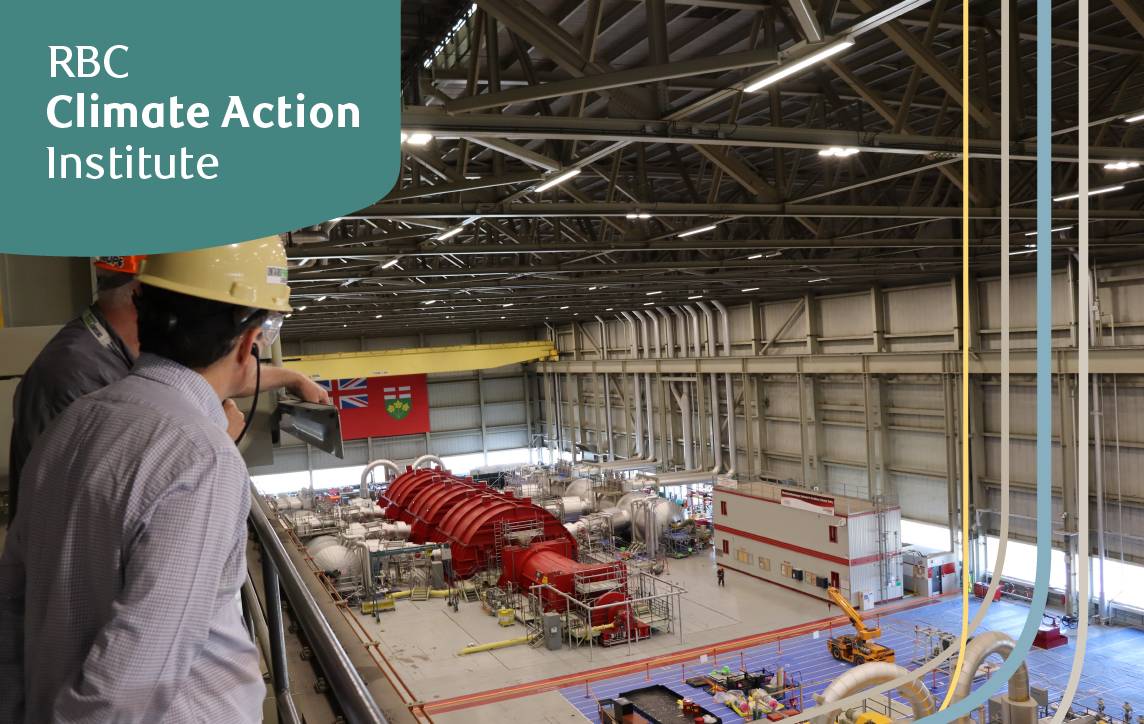
Download the Report
Contributors:
Lead author: Vivan Sorab, Senior Manager, Clean Technology
Steven Frank, Contributing editor
Caprice Biasoni, Graphic Design Specialist
- Ben Alex, Hatch
- David Dal Bello, RBC Capital Markets
- Philip Chaffee, Energy Intelligence
- George Christidis, Canadian Nuclear Association
- Lance Clarke, ARC Clean Technology
- Chris Deschenes, Ontario Power Generation
- Sara Dolatshahi, Nuclear Waste Management Organization
- John Gorman, Canadian Nuclear Association
- Frances Hilderman, Hatch
- Daniel Jurijew, Capital Power
- Dr. Chris Keefer, Canadians for Nuclear Energy
- Neal Kelly, Ontario Power Generation
- Chuck Lamers, Ontario Power Generation
- Kim Lauritsen, Ontario Power Generation
- Carlos Leipner-Gomes, LGE Strategic Advisors (Leipner Global Enterprises LLC)
- Michelle Leslie, Deloitte
- Matthew Mairinger, North American Young Generation in Nuclear
- Jon-Michael Murray, Terra Praxis
- Matthew Naraine, Canadian Nuclear Safety Commission
- Chad Richards, Nuclear Innovation Institute
- Adam Schatzker, Canada Nickel Company
- Brad Sigurdsson, Saskatchewan Mining Association
- Mathias Trojer, Prodigy Clean Energy
- James Wolf, ARC Clean Technology
- Andrew Wong, RBC Capital Markets
- Natural Resources Canada: Canada Outlines Next Steps for Progress on Small Modular Reactor Technology
- Natural Resources Canada: COP28: Declaration to Triple Nuclear Energy (2023
- Ontario Power Generation: OPG working to deploy SMR fleet to help power Ontario’s clean energy future
- Natural Resources Canada: Canadian SMR Roadmap
- Canada’s Small Modular Reactor (SMR) Action Plan
- McMaster University: McMaster Nuclear Reactor
- Nuclear facility – Royal Military College of Canada SLOWPOKE-2 research reactor
- World Nuclear Association: Small Nuclear Power Reactors
- Rolls-Royce Small Modular Reactors
- Chemical and Engineering News: Can small modular reactors at chemical plants save nuclear energy?
- The NEA Small Modular Reactor Dashboard: Second Edition
- World Nuclear Association: Small Nuclear Power Reactors
- Tennessee Valley Authority: Advanced Nuclear Solutions
- UK Research and Innovation: UK government invests £215 million into small nuclear reactors
- Natural Resources Canada: Canada Launches New Small Modular Reactor Funding Program
- Canada Infrastructure Bank: CIB commits $970 million towards Canada’s first Small Modular Reactor
- University of New Brunswick: UNB researchers are exploring how to power the future with small modular reactors
- CBC: 7 First Nations in N.B invest in small modular nuclear reactors
- Government of Saskatchewan Funds Microreactor Research
- Energy Institute: Statistical Review of World Energy 2023
- Canada Energy Regulator: Canada’s Energy Future
- RBC Climate Action Institute Analysis
- Canada Energy Regulator: Canada’s Energy Future
- RBC Climate Action Institute Analysis
- Canadian Climate Institute: Early Estimate Of National Emissions
- Nuclear Energy Agency: The NEA Small Modular Reactor Dashboard: Second Edition
- Canadian Climate Institute: Early Estimate Of National Emissions
- Electric Autonomy Canada: Vehicle orders bring Glencore’s all-electric Onaping Depth mine a step closer to fruition
- Canada Nickel: Canada Nickel Announces Carbon Storage Testing Results Better than Anticipated; Integrated Feasibility Study Expected in September
- RBC Climate Action Institute Analysis
- Canadian Climate Institute: Early Estimate Of National Emissions
- Canada Energy Regulator: Oil sands use of natural gas for production decreases considerably in early 2020
- RBC Climate Action Institute Analysis
- Lovering et al. (2016): Historical construction costs of global nuclear power reactors
- US Energy Information Administration: First new U.S. nuclear reactor since 2016 is now in operation
- POWER: A Closer Look at Two Operational Small Modular Reactor Designs
- RBC Climate Action Institute Analysis
- Canada’s Energy Future 2023: Energy Supply and Demand Projections to 2050
- RBC Climate Action Institute Analysis
- ibid
- Natural Resources Canada: Canada Supports Indigenous Advisory Council for SMR Action Plan
- CBC: 7 First Nations in N.B invest in small modular nuclear reactors
- First Nations Major Project Coalition: Primer on Nuclear Energy, SMRs and First Nations
- GE Vernova: Tennessee Valley Authority, Ontario Power Generation and Synthos Green Energy Invest in Development of GE Hitachi Small Modular Reactor Technology
- RBC Climate Action Institute analysis.
- World Nuclear Association: World Uranium Mining Production
- World Nuclear Association: Conversion and Deconversion
- BC Laws: Clean Energy Act
- Nova Scotia Legislature: Energy Reform (2024) Act
- Environics Research and Canadian Nuclear Association: Public Attitudes To Nuclear Power
- Reuters: Britain plans to boost nuclear workforce
- Fasken: A Nascent Renaissance – Part II: Confronting Nuclear Energy Fuel Supply Chain Challenges
- Ontario Power Generation: OPG selects suppliers for first fuel contracts for its Small Modular Reactors
- World Nuclear Association: Uranium Enrichment
- OPG celebrates green light for Pickering Refurbishment. Here’s what’s next
- RBC Climate Action Institute Analysis
Osler: Canada announces funding program to enable deployment of small modular reactors
This article is intended as general information only and is not to be relied upon as constituting legal, financial or other professional advice. A professional advisor should be consulted regarding your specific situation. Information presented is believed to be factual and up-to-date but we do not guarantee its accuracy and it should not be regarded as a complete analysis of the subjects discussed. All expressions of opinion reflect the judgment of the authors as of the date of publication and are subject to change. No endorsement of any third parties or their advice, opinions, information, products or services is expressly given or implied by Royal Bank of Canada or any of its affiliates.